拱線進動
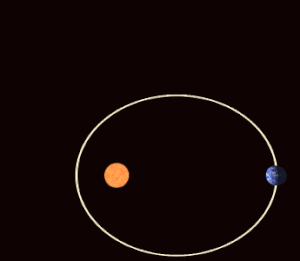


月球 · 地球
拱線進動(或拱線前進)是在天體力學中[1]連接天體軌道拱點的線(拱線)的進動(逐漸旋轉)。拱點是軌道上距離其主天體最遠(遠點)和最近(近點)的點。拱線進動是近心點幅角(近點幅角)的第一個時間導數,是軌道的六個主要軌道元素之一。當軌道軸線與軌道運動方向相同時,視為正拱線進動。拱線轉動週期是軌道前進360°所需的時間間隔[2],它使地球繞軌道運行約112,000年,完成一個週期並返回相同的方向[3]。
歷史
[编辑]古希臘天文學家喜帕恰斯注意到月球軌道的拱線進動(即月球遠地點的公轉,週期約為8.85年)[4];它在安提基特拉機械(約西元前80年)中進行了校正(假設值為8.88年/整個週期,校正到當前測量值的0.34%以內)[5]。太陽拱線的進動(作為一種不同於分點進動的運動)在二世紀由亞歷山大的托勒密首次量化。他還計算了進動對天體運動的影響[6][7][8]。地球和其它行星的拱線進動是眾多現象的結果,其中一部分一直很難解釋,直到20世紀水星拱線進動的最後一個未知部分才被準確解釋。
計算
[编辑]多種因素可能導致近星點進動,如廣義相對論、恆星四極矩、恆星-行星相互潮汐變形和其它行星的攝動[9]。
- ωtotal = ωGeneral Relativity + ωquadrupole + ωtide + ωperturbations
對於水星,由於一般相對論效應,近日點進動率為每世紀43″(弧秒)。相較之下,由於太陽系其它行星的攝動而產生的進動為每世紀532″,而太陽的扁率(四極矩)造成的貢獻可忽略不計,為每世紀0.025″[10][11]。
根據經典力學,如果恆星和行星被認為是純球形質量,那麼它們將服從一個簡單的1/r2 平方反比定律,將力與距離聯繫起來,從而根據伯特蘭定理執行閉合橢圓軌道。非球形質量效應是由外部勢能的應用引起的:旋轉體的離心勢能導致兩極之間的扁平化,附近質量的重力引起潮汐凸起。旋轉和淨潮汐凸起產生引力四極場 (1/r3)導致軌道進動。 僅考慮最低階效應,孤立的非常熱木星的總拱線進動是,並且大致按重要性順序排列
- ωtotal = ωtidal perturbations + ωGeneral Relativity + ωrotational perturbations + ωrotational * + ωtidal *
行星潮汐凸起是主導項,超過廣義相對論和恆星四極的影響超過一個數量級。潮汐凸起的良好近似結果有助於瞭解這些行星的內部。對於週期最短的行星,行星內部每年會產生幾度的進動。WASP-12b每年的角度高達19.9°[12][13]。
牛頓旋轉軌道定理
[编辑]牛頓匯出了一個早期的定理,試圖解釋拱線進動。這個定理是「歷史上」值得注意的,但它從未被廣泛使用,它提出了被發現不存在的力,使這個定理無效。直到1995年,這一旋轉軌道定理在三個多世紀的時間裏基本上一直是未知的和未開發的[14]。 牛頓提出,在不影響粒子徑向運動的情况下,粒子角運動的變化可以通過添加一個力來解釋,該力以距離的倒數變化[15]。牛頓使用泰勒級數的前身,將他的定理推廣到所有的力定律,前提是與圓形軌道的偏差很小,這對太陽系中的大多數行星都有效[來源請求]。然而,在不放棄牛頓萬有引力定律的平方反比定律的情况下,他的定理並沒有解釋月球的拱線進動。此外,通過牛頓旋轉軌道定理計算的拱線進動率不如通過攝動理論等較新方法計算的準確[來源請求]。

廣義相對論
[编辑]行星水星的近日點近動在19世紀中期被于爾班·勒威耶注意到,並由愛因斯坦的廣義相對論解釋。
1910年代,有幾個人根據狹義相對論計算了近日點的進動。他們通常獲得的值僅為正確值的1/6,即7''/年[16][17]。
愛因斯坦證明,對於一顆行星,其軌道的半長軸為{mvar|a)),軌道的離心率為{mvar|e)),公轉週期為T,那麼在弧度的一個旋轉週期內,相對論效應引起的拱線進動為
此處的c是光速[18]。以水星為例,長軸的一半大約×1010 米,其軌道離心率為0.206,公轉週期為87.97天或 5.79×106 s。從這些和光速(其值是〜 7.6×108 m/s),可以計算出一個公轉週期內的拱線進動為 3ε = ×10−7弧度 ( 5.028×10−5度或0.104″)。在一百年內,水星繞太陽旋轉約415圈,因此在這段時間內,由於相對論效應,拱線進動約為43〃,這幾乎與之前測量值中無法解釋的部分完全對應。 2.88
長期氣候
[编辑]地球的拱線進動緩慢地增加了它的近心點幅角;橢圓相對於恆星旋轉一次大約需要年 112,000[19]。相對於恆星,地球的極軸,以及至點和分點,以大約年的週期前進。這兩種形式的「歲差」結合在一起,使橢圓耗時在 26,000和 20,800年(平均而言是 29,000年)相對於春分點旋轉一次,也就是說,近日點返回到同一日期(給定一個完美跟踪季節的民用曆) 23,000[20]。
這種異常和熱帶迴圈之間的相互作用在地球上的長期氣候變化中很重要,稱為米蘭科維奇循環。米蘭科維奇循環是理解拱線進動效應的核心。 在火星也有相等的現象。
右圖說明了歲差對北半球季節的影響,相對於近日點和遠日點。 請注意,在特定季節掃過的區域會隨時間變化。軌道力學要求季節的長度與季節象限的掃掠面積成比例,因此當軌道離心率極端時,軌道遠端的季節持續時間可能會更長。

相關條目
[编辑]- 軸向進動
- 節點進動
- 下擺線
- 羅塞塔(軌道)
- 螺旋描記器
註解
[编辑]- ^ Bowler, M. G. Apsidal advance in SS 433?. Astronomy and Astrophysics. 2010, 510 (1): A28. Bibcode:2010A&A...510A..28B. S2CID 119289498. arXiv:0910.3536
. doi:10.1051/0004-6361/200913471.
- ^ Hilditch, R. W. An Introduction to Close Binary Stars. Cambridge astrophysics series. Cambridge University Press. 2001: 132. ISBN 9780521798006.
- ^ Buis, Alan; Laboratory, s Jet Propulsion. Milankovitch (Orbital) Cycles and Their Role in Earth's Climate – Climate Change: Vital Signs of the Planet. Climate Change: Vital Signs of the Planet. 2020-02-27 [2023-06-02].
- ^ Jones, A., Alexander. The Adaptation of Babylonian Methods in Greek Numerical Astronomy (PDF). Isis. September 1991, 82 (3): 440–453 [7 August 2014]. Bibcode:1991Isis...82..441J. S2CID 92988054. doi:10.1086/355836. (原始内容 (PDF)存档于4 March 2016).
- ^ Freeth, Tony; Bitsakis, Yanis; Moussas, Xenophon; Seiradakis, John. H.; Tselikas, A.; Mangou, H.; Zafeiropoulou, M.; Hadland, R.; et al. Decoding the ancient Greek astronomical calculator known as the Antikythera Mechanism (PDF). Nature. 30 November 2006,. 444 Supplement (7119): 587–91 [20 May 2014]. Bibcode:2006Natur.444..587F. PMID 17136087. S2CID 4424998. doi:10.1038/nature05357. (原始内容 (PDF)存档于20 July 2015).
- ^ Toomer, G. J., The Solar Theory of az-Zarqāl: A History of Errors, Centaurus, 1969, 14 (1): 306–336, Bibcode:1969Cent...14..306T, doi:10.1111/j.1600-0498.1969.tb00146.x, at pp. 314–317.
- ^ Ptolemaic Astronomy in the Middle Ages. princeton.edu. [21 October 2022].
- ^ C. Philipp E. Nothaft. Criticism of trepidation models and advocacy of uniform precession in medieval Latin astronomy. Archive for History of Exact Sciences. 2017, 71 (3): 211–244. S2CID 253894382. doi:10.1007/s00407-016-0184-1.
- ^ David M. Kipping. The Transits of Extrasolar Planets with Moons. Springer. 8 August 2011: 84– [27 August 2013]. ISBN 978-3-642-22269-6.
- ^ Kane, S. R.; Horner, J.; von Braun, K. Cyclic Transit Probabilities of Long-period Eccentric Planets due to Periastron Precession. The Astrophysical Journal. 2012, 757 (1): 105. Bibcode:2012ApJ...757..105K. S2CID 54193207. arXiv:1208.4115
. doi:10.1088/0004-637x/757/1/105.
- ^ Richard Fitzpatrick. An Introduction to Celestial Mechanics. Cambridge University Press. 30 June 2012: 69 [26 August 2013]. ISBN 978-1-107-02381-9.
- ^ Ragozzine, D.; Wolf, A. S. Probing the interiors of very hot Jupiters using transit light curves. The Astrophysical Journal. 2009, 698 (2): 1778–1794. Bibcode:2009ApJ...698.1778R. S2CID 29915528. arXiv:0807.2856
. doi:10.1088/0004-637x/698/2/1778.
- ^ Michael Perryman. The Exoplanet Handbook. Cambridge University Press. 26 May 2011: 133– [26 August 2013]. ISBN 978-1-139-49851-7.
- ^ Chandrasekhar, p. 183.
- ^ Lynden-Bell, D.; Jin, S. Analytic central orbits and their transformation group. Monthly Notices of the Royal Astronomical Society. 1 May 2008, 386 (1): 245–260. Bibcode:2008MNRAS.386..245L. ISSN 0035-8711. S2CID 15451037. arXiv:0711.3491
. doi:10.1111/j.1365-2966.2008.13018.x.
- ^ McDonald, Kirk T. "Special Relativity and the Precession of the Perihelion." JH Lab., Princeton University (2023).
- ^ Goldstein, Herbert; Poole, Charles P.; Safko, John L. Classical mechanics 3. ed., [Nachdr.] San Francisco Munich: Addison Wesley. 2008. Chapter 7, Exercise 27, Page 332. ISBN 978-0-201-65702-9.
- ^ Hawking, Stephen. On the Shoulders of Giants : the Great Works of Physics and Astronomy. Philadelphia, Pennsylvania, USA: Running Press. 2002: der Physik. ISBN 0-7624-1348-4.
- ^ van den Heuvel, E. P. J. On the Precession as a Cause of Pleistocene Variations of the Atlantic Ocean Water Temperatures. Geophysical Journal International. 1966, 11 (3): 323–336. Bibcode:1966GeoJ...11..323V. doi:10.1111/j.1365-246X.1966.tb03086.x
.
- ^ The Seasons and the Earth's Orbit, United States Naval Observatory, [16 August 2013], (原始内容存档于2 August 2013)
Text is available under the CC BY-SA 4.0 license; additional terms may apply.
Images, videos and audio are available under their respective licenses.