吸积

在天文物理學中,吸積是指大質量物體透過其重力吸引更多物質(大多為氣態物質)進入吸積盤,進而使粒子積聚的過程。[1][2]大多數天體,諸如星系、恆星與行星等,均是藉由吸積的過程形成。
簡介
[编辑]主張地球與其他類地行星均是由流星體構成的吸積模型,最早由奧托·施密特於1944年提出,並由威廉·麥克雷(1960)的原行星理論與邁克爾·沃爾夫森的捕捉理論承繼。[3] 1978年,安德魯·普倫蒂斯重拾最初拉普拉斯對於行星形成之想法,並發展出「近代拉普拉斯理論」。[3]上述理論均非完全成功,且許多學說僅是描述性的。
奧托·施密特於1944年提出的吸積模型在1969年被維克托·薩夫羅諾夫發展為定量描述之形式。[4] 他詳細計算了類地行星形成的各個階段。[5][6]自此,透過深入的數值模擬,此一模型在微行星形成之研究上獲得發展。現今,恆星因星際氣體的重力塌縮而形成的概念已被接受。在塌縮之前,這些氣體最主要以分子雲的形式存在,例如獵戶座星雲。隨著分子雲塌縮,位能減少,進而使其溫度、動能均增加,角動量守恆確定分子雲將形成一扁平圓盤—也就是吸積盤。
星系的吸積
[编辑]大霹靂後的數十萬年後,宇宙已冷卻到原子得以形成。隨著宇宙持續膨脹並冷卻,原子失去足夠的動能,暗物質充分合成,進而形成原星系。星系隨著進一步的吸積而形成。[7]非直接性的證據十分普遍。[7]星系藉由碰撞合併成長,並使氣體吸積得以平整、順暢。吸積亦會於星系內部發生,並形成恆星。
恆星的吸積
[编辑]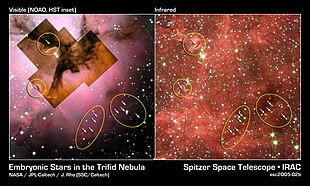
恆星被認為是在由低溫氫分子構成,質量約為300,000 M☉,直徑約65光年(20秒差距)的巨型分子雲內部形成。[8][9]數百萬年之後,巨型分子雲容易發生塌縮並碎裂。[10]其產生的碎片接著形成小巧但緻密的核,這些核最後將塌縮為恆星。[9]其質量範圍為不足一倍太陽質量至數倍太陽質量,並且被稱作原恆星(原太陽)星雲。[8]其直徑介於2000至20000天文單位(0.01至0.1秒差距),粒子密度約介於每10000至100000立方公分(16000至160000立方英吋)一個粒子。請將之與海平面處空氣的粒子密度—每×1019立方公分( 2.8×1020立方英吋)一個分子—比較。 4.6[9][11]
質量與太陽相近的原星雲,最初的塌縮約耗時100,000年。[8][9]一開始,每個星雲都具有一定的角動量。星雲中心的氣體帶有較少角動量,經歷急速的壓縮,形成一高溫、達流體靜力平衡的(非壓縮的)核心,其內包含原本星雲的一小部分質量。這個核「種下」了即將成為恆星的種子。[8]隨著塌縮繼續進行,根據角動量守恆,往內落入的包層將加速旋轉,最終形成一圓盤狀結構。

隨著物質持續墜入圓盤,包層逐漸變得薄而透明,而可觀測到年輕恆星體(young stellar object,YSO),最初可見於遠紅外線波段,而後來到可見光波段。[11]此時,原恆星開始融合生成氘。若原恆星的質量夠大(大於80 MJ) ,氫融合將接續發生。相反的,若其質量過小,這些物體將成為棕矮星。[12]恆星的誕生約發生於塌縮開始後的100,000年。[8]此階段的物體稱為第一型原恆星,又被稱作年輕金牛T星、經演化的原恆星、年輕恆星體等。此時,形成中的恆星已吸積其大部分質量;吸積盤與其他包層的總質量不超過中心YSO的百分之10至20。[11]

在下一個階段,包層被吸積盤聚集並完全消失,此時原恆星成為典型的金牛T星。[13]後者具有吸積盤並持續吸聚高溫氣體,此一現象可見於其光譜中的強放射譜線。前者不具有吸積盤。 典型金牛T星演化成為譜線強度較弱的金牛T星。[14]這大約發生於一百萬年後。[8]環繞著一顆典型金牛T星的吸積盤之質量,約為該恆星本身質量的1–3%,且其約以每年10−7 至10−9 M☉的速率吸積物質。[15]與此同時,通常也會出現一對雙極噴流。吸積可以解釋各種金牛T星的古怪現象:光譜線的極大通量(最大可達其固有光度的100%)、磁性活動、測得光度的變動性與噴流等。[16]實際上,放射譜線形成於吸積氣體碰撞到恆星的「表面」,這發生於其磁極附近。[16]噴流是吸積的副產物,帶走多餘的角動量。典型金牛T星的階段約可維持一千萬年。[8]這僅有少數例外,例如所謂的彼得潘吸積盤,其吸積時間超過二千萬年。[17]吸積盤最後將因被吸入中心恆星、行星形成、被噴流射出、來自中心或周圍恆星的紫外輻射造成的光致蒸發等而消失。[18]因此,這顆年輕的恆星將成為一顆譜線強度較弱的金牛T星譜線強度較弱的金牛T星,並在數億年之後,演化成為一顆類似太陽的普通恆星,其細節取決於恆星的初始質量。
行星的吸積
[编辑]
宇宙塵埃的自我吸積加速粒子墜入巨石尺寸的微行星。質量較大的小行星會吸積質量較小者,質量較小者則在碰撞中碎裂。吸積盤常見於較小恆星、密近雙星的恆星殘餘物,或被物質環繞的黑洞(諸如位於星系中心的黑洞)等的周圍。 環繞的氣體損失角動量並墜入中心處的大質量物體的過程,仰賴於吸積盤中的某些動力,例如動態摩擦。在某些情況下,這可能由恆星表面核融合造成(參見邦迪吸積)。
在類地行星或行星核的形成過程中,我們考慮以下幾個階段。首先,當氣體與灰塵顆粒碰撞,他們如同凡得瓦力和電磁作用力般,經由微觀物理的過程凝聚,並形成毫米尺度的顆粒;於此階段,積累的機制大體上是非重力的。[19]然而,微行星在公分至公尺尺度的形成尚未被完整了解,亦無具說服力的說法能解釋為核這些顆粒會積聚而非直接反彈。[19]:341特別地,至今仍不清楚這些物體如何成為0.1至1公里(0.06至0.6英里)大小的微行星;[5][20]此一問題被稱作「公尺尺度障礙」:[21][22] 當灰塵粒子凝集成長,它們獲得了相對於附近其他粒子越來越大的相對速度與系統的向內漂移速度,並導致其發生破壞性的碰撞,也因此限制其凝聚不得超過某一最大尺寸。[23]華德(1996)提出當速度慢的顆粒發生碰撞,他們之間極小但非零的重力阻止其脫離。[19]:341 顆粒的碎片化亦被認為在補充微小顆粒、保持吸積盤厚度,甚至是使各種尺寸固體均維持一相對高的豐度上,均扮演重要角色。[23]
天文學家提出了許多機制,試圖跨越「公尺尺度障礙」。 石塊間可能形成區域性的聚集,接著受重力而塌縮成巨大小行星尺度的微行星。這些聚集可能因為氣體吸積盤的結構而被動地發生,例如:在渦流中、於壓力撞擊處、在巨行星製造的縫隙邊緣處、或在吸積盤的急流區域邊緣。[24]抑或者,微粒可能在積聚過程中透過稱為沖流不穩性的回饋機制扮演積極的角色。在沖流不穩性中,原行星吸積盤中固體與氣體的交互作用將導致區域性的凝聚成長,新的微粒累積在小規模凝聚處,使其成為大質量的細絲。[24] 相反的,若灰塵凝聚形成的顆粒具高度滲透性,其成長可能持續直到其質量足以使其因自身引力而塌縮。這些物質的低密度使其能和氣體保持高度連結,也因此避免了可能導致侵蝕或破碎的高速。[25]
顆粒間最終將相互黏附,形成尺寸與山相若(或更大)的物體,稱作微行星。碰撞與微行星間的重力交互作用結合,在約十至一百萬年後產生月球尺寸的行星胚胎原行星。 最終,在一千萬至一億年後,行星胚胎間相互碰撞,形成行星。[20]這些微行星的質量足夠大,使得在計算其演化時,彼此間的重力交互作用足夠顯著而會被納入考量。[5]受氣體拖曳,其成長受益於繞行軌道的衰減,避免其在不同行星胚胎的軌道間「擱淺」。[26][27]更進一步的碰撞及積聚產生了類地行星或巨行星的核心。
若微行星因區域性石塊凝集的重力塌縮而形成,這些石塊的進一步吸積將主導微行星成長為行星胚胎及巨行星核心的過程。石塊的吸積受益於向大質量物體加速時,物體感受到的氣體拖曳。氣體拖曳使石塊速度減低至大質量物體的脫離速度以下,使其向該物體旋轉而下並被其吸積。石塊的吸積可能使行星的形成相較於微行星的吸積加速1000倍,使得巨行星能在氣體吸積盤耗散前形成。[28][29] 然而,核心透過石塊吸積的成長與天王星與海王星的最終質量和組成無法吻合。[30]
類地行星的形成與氣體巨行星(又被稱為類木行星)有所不同。組成類地行星的粒子由太陽系內部壓密的金屬及岩石組成。然而,類木行星起始於巨大而冰封的微行星,這些微行星自太陽星雲捕捉氫與氦。[31] 此二類別微行星的區分乃根據太陽星雲的凍線。[32]
小行星的吸積
[编辑]
隕石內含有小行星起源與演化各階段中,吸積、受撞擊等的記錄;然而,其吸積與成長的機制尚未被完全了解。[33]證據顯示小行星的主要成長可能是球粒隕石受氣體輔助而吸積的結果,這些隕石是毫米尺度的小球,在被吸積至其母小行星前以熔融(或部分熔融)的液滴形態存在於太空中。[33]在內太陽系,球粒隕石被發現在啟動吸積上至關重要。[34]小行星的微小質量可能部分是因為2AU外球狀隕石的形成不足,或是因為原恆星附近球狀隕石的輸送效率較低。[34]此外,撞擊控制了小行星的形成與毀滅,並被認為在其地質演化上是一個主要影響因子。[34]
球粒隕石、金屬顆粒與其他成分很可能於太陽星雲中形成。 他們吸積在一起,形成母小行星。這些物體中的一部份接著熔化,形成金屬核心與富含橄欖石的地幔;其他則發生水性的改變。[34]在小行星冷卻後的45億年內,他們將因撞擊而被侵蝕,甚至瓦解。[35]
為使吸積得以發生,撞擊速度約須小於其脫離速度的二倍,對於一個半徑100公里(60英里)的小行星,其值約為140公尺/秒(460英尺/秒)。[34]小行星帶的簡易的吸積模型通常假設微米尺寸的灰塵顆粒相互黏附並落於星雲的半平面,形成一灰塵的密集層;由於重力,此層被轉換為公里尺寸微行星的吸積盤。然而,不少論述指出小行星可能並非以此方式吸積。[34]
彗星的吸積
[编辑]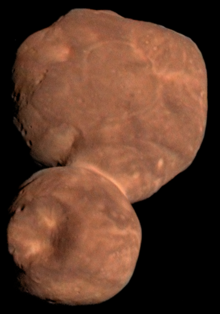
彗星,或其前身,形成於外太陽系,這可能發生在行星形成的數百萬年前。[36]彗星形成的時間與方式備受爭論,這對於太陽系形成、動力學與地質學都有清楚的含義。三維電腦模擬指出彗核上觀測到的主要結構特徵可以被微弱彗星成對的低速吸積所解釋。[37][38] 現今較偏好的形成機制是星雲假說,此機制認為彗星可能是最初原行星(行星由其成長而成)「組成部件」的殘餘物。[39][40][41]
天文學家認為彗星源自於歐特雲與離散盤。[42] 離散盤形成於海王星向外遷移至原庫伯帶(此時其與太陽的距離近很多)時,並在其其尾流區留下動力穩定,可能永遠不會被其軌道(庫伯帶性質)影響的物體,以及另一些近日點距離海王星夠近,仍會在繞太陽行進時被海王星干擾的物體(離散盤)。由於離散盤及庫伯帶分別處於動力活躍與相對動力穩定的狀態,現今離散盤被認為是週期性彗星最可能的起源處。[42]古典歐特雲理論宣稱歐特雲,一個半徑約50000 天文單位(0.24秒差距)的球,與太陽星雲同時形成,並有時會在巨行星或恆星經過其附近造成重力干擾時,釋出彗星至內太陽系。[43]這類彗星雲可能已被見於螺旋星雲。[44]
2015年,前往彗星丘留莫夫-格拉西緬科彗星的「羅塞塔」任務,確定了當太陽的熱穿透其表層,將引發埋藏的冰的汽化(昇華)。少部份產生的水蒸氣可能自彗核脫離,但百分之80於其表面下的層中再次凝固。[45]這項觀測暗示暴露於表層附近富含冰的薄層可能是彗星活動與演化的結果,且全體性的分層並不必然發生於彗星形成歷史的早期。[45][46]儘管大多數科學家認為所有證據都顯示彗核的結構是經前一世代較小的冰封微行星處理過的碎石堆[47] 「羅塞塔」任務打消了彗星是截然不同的材料形成的「碎石堆」之想法。[48][49]
參見
[编辑]參考
[编辑]- ^ Science with the VLTI. European Southern Observatory. 2008-08-08 [2011-04-11]. (原始内容存档于2011-05-24).
- ^ Masters, Harris. Transcript of The Accretion of Galaxies and Stars. Prezi. 2010-08-26 [2016-01-08].
- ^ 3.0 3.1 Woolfson, M. M. The Solar System—its Origin and Evolution. Quarterly Journal of the Royal Astronomical Society. March 1993, 34: 1–20. Bibcode:1993QJRAS..34....1W.
For details of Kant's position, see Palmquist, Stephen. Kant's Cosmogony Re-evaluated. Studies in History and Philosophy of Science. September 1987, 18 (3): 255–269. Bibcode:1987SHPS...18..255P. doi:10.1016/0039-3681(87)90021-5. - ^ Henbest, Nigel. Birth of the planets: The Earth and its fellow planets may be survivors from a time when planets ricocheted around the Sun like ball bearings on a pinball table. New Scientist. 1991-08-24 [2008-04-18]. (原始内容存档于2020-07-25).
- ^ 5.0 5.1 5.2 Papaloizou, John C. B.; Terquem, Caroline. Planet formation and migration (PDF). CERN. 2005-11-28 [2015-10-21]. (原始内容存档 (PDF)于2020-05-09).
- ^ Safronov, Viktor S. Evolution of the Protoplanetary Cloud and Formation of the Earth and the Planets. Jerusalem: Israel Program for Scientific Translations. 1972 [1969]. ISBN 0-7065-1225-1. hdl:2027/uc1.b4387676. NASA Technical Translation F-677.
- ^ 7.0 7.1 Kereš, Dušan; Davé, Romeel; Fardal, Mark; Faucher-Giguere, C.-A.; Hernquist, Lars; et al. Gas Accretion in Galaxies (PDF). Massive Galaxies Over Cosmic Time 3. 8–10 November 2010. Tucson, Arizona. National Optical Astronomy Observatory. 2010 [2022-05-15]. (原始内容存档 (PDF)于2017-05-19).
- ^ 8.0 8.1 8.2 8.3 8.4 8.5 8.6 Montmerle, Thierry; Augereau, Jean-Charles; Chaussidon, Marc; Counelle, Mathieu; Marty, Bernard; et al. Solar System Formation and Early Evolution: the First 100 Million Years. Earth, Moon, and Planets. June 2006, 98 (1–4): 39–95. Bibcode:2006EM&P...98...39M. S2CID 120504344. doi:10.1007/s11038-006-9087-5.
- ^ 9.0 9.1 9.2 9.3 Pudritz, Ralph E. Clustered Star Formation and the Origin of Stellar Masses. Science. January 2002, 295 (5552): 68–75. Bibcode:2002Sci...295...68P. PMID 11778037. S2CID 33585808. doi:10.1126/science.1068298.
- ^ Clark, Paul C.; Bonnell, Ian A. The onset of collapse in turbulently supported molecular clouds. Monthly Notices of the Royal Astronomical Society. July 2005, 361 (1): 2–16. Bibcode:2005MNRAS.361....2C. doi:10.1111/j.1365-2966.2005.09105.x
.
- ^ 11.0 11.1 11.2 Motte, F.; Andre, P.; Neri, R. The initial conditions of star formation in the ρ Ophiuchi main cloud: wide-field millimeter continuum mapping. Astronomy and Astrophysics. August 1998, 336: 150–172. Bibcode:1998A&A...336..150M.
- ^ Stahler, Steven W. Deuterium and the Stellar Birthline. The Astrophysical Journal. September 1988, 332: 804–825. Bibcode:1988ApJ...332..804S. doi:10.1086/166694.
- ^ Mohanty, Subhanjoy; Jayawardhana, Ray; Basri, Gibor. The T Tauri Phase down to Nearly Planetary Masses: Echelle Spectra of 82 Very Low Mass Stars and Brown Dwarfs. The Astrophysical Journal. June 2005, 626 (1): 498–522. Bibcode:2005ApJ...626..498M. S2CID 8462683. arXiv:astro-ph/0502155
. doi:10.1086/429794.
- ^ Martin, E. L.; Rebolo, R.; Magazzu, A.; Pavlenko, Ya. V. Pre-main sequence lithium burning. Astronomy and Astrophysics. February 1994, 282: 503–517. Bibcode:1994A&A...282..503M. arXiv:astro-ph/9308047
.
- ^ Hartmann, Lee; Calvet, Nuria; Gullbring, Eric; D'Alessio, Paula. Accretion and the evolution of T Tauri disks. The Astrophysical Journal. March 1998, 495 (1): 385–400. Bibcode:1998ApJ...495..385H. doi:10.1086/305277
.
- ^ 16.0 16.1 Muzerolle, James; Calvet, Nuria; Hartmann, Lee. Emission-line diagnostics of T Tauri magnetospheric accretion. II. Improved model tests and insights into accretion physics. The Astrophysical Journal. April 2001, 550 (2): 944–961. Bibcode:2001ApJ...550..944M. doi:10.1086/319779
.
- ^ Silverberg, Steven M.; Wisniewski, John P.; Kuchner, Marc J.; Lawson, Kellen D.; Bans, Alissa S.; Debes, John H.; Biggs, Joseph R.; Bosch, Milton K. D.; Doll, Katharina; Luca, Hugo A. Durantini; Enachioaie, Alexandru. Peter Pan Disks: Long-lived Accretion Disks Around Young M Stars. The Astrophysical Journal. 2020-01-14, 890 (2): 106. Bibcode:2020ApJ...890..106S. S2CID 210718358. arXiv:2001.05030
. doi:10.3847/1538-4357/ab68e6.
- ^ Adams, Fred C.; Hollenbach, David; Laughlin, Gregory; Gorti, Uma. Photoevaporation of circumstellar disks due to external far-ultraviolet radiation in stellar aggregates. The Astrophysical Journal. August 2004, 611 (1): 360–379. Bibcode:2004ApJ...611..360A. S2CID 16093937. arXiv:astro-ph/0404383
. doi:10.1086/421989.
- ^ 19.0 19.1 19.2 Ward, William R. Planetary Accretion. ASP Conference Series. Completing the Inventory of the Solar System. 1996, 107: 337–361. Bibcode:1996ASPC..107..337W.
- ^ 20.0 20.1 Chambers, John E. Planetary accretion in the inner Solar System. Earth and Planetary Science Letters. July 2004, 233 (3–4): 241–252. Bibcode:2004E&PSL.223..241C. doi:10.1016/j.epsl.2004.04.031.
- ^ Küffmeier, Michael. What is the meter size barrier?. Astrobites. 2015-04-03 [2015-01-15]. (原始内容存档于2015-04-07).
- ^ Grishin, Evgeni; et al. Planet seeding through gas-assisted capture of interstellar objects. Monthly Notices of the Royal Astronomical Society. August 2019, 487 (3): 3324–3332. Bibcode:2019MNRAS.487.3324G. arXiv:1804.09716
. doi:10.1093/mnras/stz1505.
- ^ 23.0 23.1 Birnstiel, T.; Dullemond, C. P.; Brauer, F. Dust retention in protoplanetary disks. Astronomy and Astrophysics. August 2009, 503 (1): L5–L8. Bibcode:2009A&A...503L...5B. S2CID 12932274. arXiv:0907.0985
. doi:10.1051/0004-6361/200912452.
- ^ 24.0 24.1 Johansen, A.; Blum, J.; Tanaka, H.; Ormel, C.; Bizzarro, M.; Rickman, H. The Multifaceted Planetesimal Formation Process. Beuther, H.; Klessen, R. S.; Dullemond, C. P.; Henning, T. (编). Protostars and Planets VI. University of Arizona Press. 2014: 547–570. Bibcode:2014prpl.conf..547J. ISBN 978-0-8165-3124-0. S2CID 119300087. arXiv:1402.1344
. doi:10.2458/azu_uapress_9780816531240-ch024.
- ^ Johansen, A.; Jacquet, E.; Cuzzi, J. N.; Morbidelli, A.; Gounelle, M. New Paradigms For Asteroid Formation. Michel, P.; DeMeo, F.; Bottke, W. (编). Asteroids IV. Space Science Series. University of Arizona Press. 2015: 471. Bibcode:2015aste.book..471J. ISBN 978-0-8165-3213-1. S2CID 118709894. arXiv:1505.02941
. doi:10.2458/azu_uapress_9780816532131-ch025.
- ^ Weidenschilling, S. J.; Spaute, D.; Davis, D. R.; Marzari, F.; Ohtsuki, K. Accretional Evolution of a Planetesimal Swarm. Icarus. August 1997, 128 (2): 429–455. Bibcode:1997Icar..128..429W. doi:10.1006/icar.1997.5747.
- ^ Kary, David M.; Lissauer, Jack; Greenzweig, Yuval. Nebular Gas Drag and Planetary Accretion. Icarus. November 1993, 106 (1): 288–307. Bibcode:1993Icar..106..288K. doi:10.1006/icar.1993.1172.
- ^ Lewin, Sarah. To Build a Gas Giant Planet, Just Add Pebbles. Space.com. 2015-08-19 [2015-11-22]. (原始内容存档于2023-02-23).
- ^ Lambrechts, M.; Johansen, A. Rapid growth of gas-giant cores by pebble accretion. Astronomy & Astrophysics. August 2012, 544: A32. Bibcode:2012A&A...544A..32L. S2CID 53961588. arXiv:1205.3030
. doi:10.1051/0004-6361/201219127.
- ^ Helled, Ravit; Bodenheimer, Peter. The Formation of Uranus and Neptune: Challenges and Implications for Intermediate-mass Exoplanets. The Astrophysical Journal. July 2014, 789 (1). 69. Bibcode:2014ApJ...789...69H. S2CID 118878865. arXiv:1404.5018
. doi:10.1088/0004-637X/789/1/69.
- ^ D'Angelo, Gennaro; Durisen, Richard H.; Lissauer, Jack J. Giant Planet Formation. Seager, Sara (编). Exoplanets. University of Arizona Press. December 2010: 319–346. Bibcode:2010exop.book..319D. ISBN 978-0-8165-2945-2. arXiv:1006.5486
.
- ^ Bennett, Jeffrey; Donahue, Megan; Schneider, Nicholas; Voit, Mark. Formation of the Solar System. The Cosmic Perspective 7th. San Francisco: Pearson. 2014: 136–169. ISBN 978-0-321-89384-0.
- ^ 33.0 33.1 Johansen, Anders. Growth of asteroids, planetary embryos, and Kuiper belt objects by chondrule accretion. Science Advances. April 2015, 1 (3): e1500109. Bibcode:2015SciA....1E0109J. PMC 4640629
. PMID 26601169. arXiv:1503.07347
. doi:10.1126/sciadv.1500109.
- ^ 34.0 34.1 34.2 34.3 34.4 34.5 Scott, Edward R. D. Meteorite Evidence for the Accretion and Collisional Evolution of Asteroids (PDF). Bottke Jr., W. F.; Cellino, A.; Paolicchi, P.; Binzel, R. P. (编). Asteroids III. University of Arizona Press. 2002: 697–709 [2022-05-15]. Bibcode:2002aste.book..697S. ISBN 978-0-8165-2281-1. (原始内容存档 (PDF)于2021-06-27).
- ^ Shukolyukov, A.; Lugmair, G. W. Chronology of Asteroid Accretion and Differentiation (PDF). Bottke Jr., W. F.; Cellino, A.; Paolicchi, P.; Binzel, R. P. (编). Asteroids III. 2002: 687–695 [2022-05-15]. Bibcode:2002aste.book..687S. ISBN 978-0-8165-2281-1. (原始内容存档 (PDF)于2022-03-04).
- ^ How comets were assembled. University of Bern, via Phys.org. 2015-05-29 [2016-01-08]. (原始内容存档于2023-03-12).
- ^ Jutzi, M.; Asphaug, E. The shape and structure of cometary nuclei as a result of low-velocity accretion. Science. June 2015, 348 (6241): 1355–1358. Bibcode:2015Sci...348.1355J. PMID 26022415. S2CID 36638785. doi:10.1126/science.aaa4747.
- ^ Weidenschilling, S. J. The Origin of Comets in the Solar Nebula: A Unified Model. Icarus. June 1997, 127 (2): 290–306. Bibcode:1997Icar..127..290W. doi:10.1006/icar.1997.5712.
- ^ Choi, Charles Q. Comets: Facts About The 'Dirty Snowballs' of Space. Space.com. 2014-11-15 [2016-01-08]. (原始内容存档于2021-09-22).
- ^ Nuth, Joseph A.; Hill, Hugh G. M.; Kletetschka, Gunther. Determining the ages of comets from the fraction of crystalline dust. Nature. 2000-07-20, 406 (6793): 275–276. Bibcode:2000Natur.406..275N. PMID 10917522. S2CID 4430764. doi:10.1038/35018516.
- ^ How Asteroids and Comets Formed. Science Clarified. [2016-01-16]. (原始内容存档于2023-02-05).
- ^ 42.0 42.1 Levison, Harold F.; Donnes, Luke. Comet Populations and Cometary Dynamics. McFadden, Lucy-Ann Adams; Weissman, Paul Robert; Johnson, Torrence V. (编). Encyclopedia of the Solar System 2nd. Amsterdam: Academic Press. 2007: 575–588. ISBN 978-0-12-088589-3.
- ^ Greenberg, Richard. The Origin of Comets among the Accreting Outer Planets. Carusi, Andrea; Valsecchi, Giovanni B. (编). Dynamics of Comets: Their Origin and Evolution. Astrophysics and Space Science Library, Volume 115 115. Springer Netherlands. 1985: 3–10. Bibcode:1985ASSL..115....3G. ISBN 978-94-010-8884-8. doi:10.1007/978-94-009-5400-7_1.
- ^ Evaporation and Accretion of Extrasolar Comets Following White Dwarf Kicks. Cornell University Department of Astronomy. 2014 [2016-01-22]. (原始内容存档于2018-06-17).
- ^ 45.0 45.1 Filacchione, Gianrico; Capaccioni, Fabrizio; Taylor, Matt; Bauer, Markus. Exposed ice on Rosetta's comet confirmed as water (新闻稿). European Space Agency. 2016-01-13 [2016-01-14]. (原始内容存档于2016-01-18).
- ^ Filacchione, G.; de Sanctis, M. C.; Capaccioni, F.; Raponi, A.; Tosi, F.; et al. Exposed water ice on the nucleus of comet 67P/Churyumov–Gerasimenko. Nature. 2016-01-13, 529 (7586): 368–372. Bibcode:2016Natur.529..368F. PMID 26760209. S2CID 4446724. doi:10.1038/nature16190.
- ^ Krishna Swamy, K. S. Physics of Comets. World Scientific Series in Astronomy and Astrophysics, Volume 2 2nd. World Scientific. May 1997: 364. ISBN 981-02-2632-2.
- ^ Khan, Amina. After a bounce, Rosetta. Los Angeles Times. 2015-07-31 [2016-01-22]. (原始内容存档于2018-11-06).
- ^ Rosetta's frequently asked questions. European Space Agency. 2015 [2016-01-22]. (原始内容存档于2012-12-17).
|
Text is available under the CC BY-SA 4.0 license; additional terms may apply.
Images, videos and audio are available under their respective licenses.